Soybean Soil Fertility
(SF1164, Revised Nov. 2019)All of NDSU soil fertility recommendations now have no yield-based formulas. The soybean fertility recommendations were modified to be in line with these new guidelines.
R. Jay Goos, Professor Soil Science Department; Hans Kandel, Extension Agronomist; Chris Augustin, Extension Soil Health Specialist (North Central Research Extension Center); Ryan Buetow, Extension Cropping Systems Specialist(Dickinson Research Extension Center); Jasper Teboh, Extension Soil Scientist (NDSU Carrington Research Extension Center); Shana Forster, Director(NDSU North Central Research Extension Center); Greg Endres, Extension Cropping Systems Specialist (Carrington Research Extension Center)
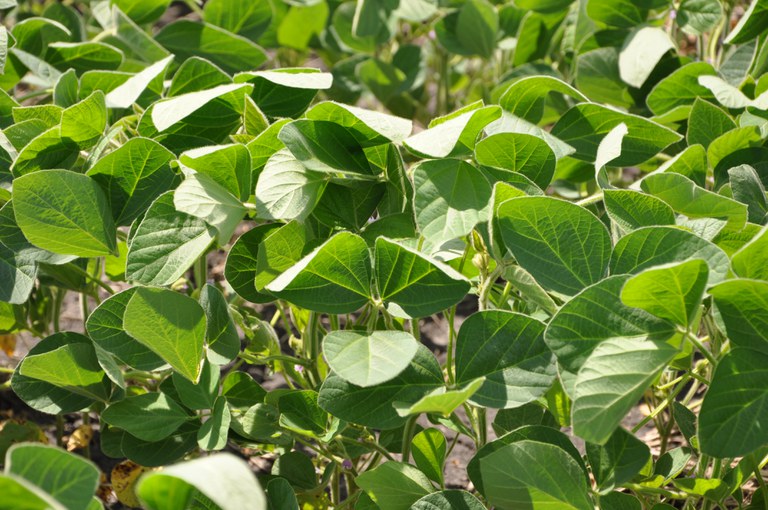
Soybeans require 14 mineral nutrients: nitrogen (N), phosphorus (P), potassium (K), sulfur (S), calcium (Ca), magnesium (Mg), copper (Cu), iron (Fe), manganese (Mn), zinc (Zn), boron (B), chloride (Cl), molybdenum (Mo) and nickel (Ni) to grow successfully.
North Dakota soils provide adequate amounts for soybean production except for N, P, K, S and Fe.
Nitrogen
Nodulation
Although the atmosphere is 78% nitrogen (N) gas, plants cannot use it directly. Plants can use only ammonium-N or nitrate-N. Soybeans are a legume and normally should provide adequate N through a symbiotic relationship with N-fixing bacteria of the species Bradyrhizobium japonicum. In this symbiotic relationship, carbohydrates and minerals are supplied to the bacteria by the plant, and the bacteria transform nitrogen gas from the atmosphere into ammonium-N for use by the plant.
The process of soybean infection by N-fixing bacteria and symbiotic N fixation is a complex process between the bacteria and the plant. The correct species of N-fixing bacteria must be present in the soil, either through inoculation of the seed or the seed zone at planting.
Nitrogen-fixing bacteria are attracted to soybean roots by chemical signals from the soybean root in the form of flavenoid compounds (1). Once in contact with the root hairs, a root compound binds the bacteria to the root hair cell wall. The bacteria releases a chemical that causes curling and cracking of the root hair, allowing the bacteria to invade the interior of the cell and begin to change the plant cell structure to form nodules (2, 3, 4) (Figure 1, Page 2).
The bacteria live in compartments, up to 10,000 in each nodule, called bacteroids (Figure 2, Page 3). Each bacteroid is bathed in nutrients from the host plant, and the bacteroid takes N2 gas from the soil air and converts it to ammonium-N using the enzyme nitrogenase, which consists of one Fe-Mo (iron-molybdenum)-based protein and two Fe (iron)-based proteins. Iron deficiency chlorosis (IDC) may result in poor nodulation.

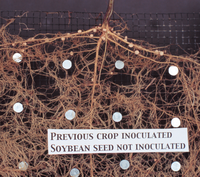
Inoculation
If soybean will be planted in a field for the first time, the seed will need to be inoculated with Bradyrhizobium japonicum (soybean inoculum). Several inoculums types can be used: peat-based, liquid-based or granular.
Of the three, granular appears to be the most fool-proof for a first inoculation. The other two also can be used, but the frequency of mistakes is much higher. No formulation is free of error.
For the peat-based and liquid-based treatments, all seed should have inoculum attached to it when it enters the soil. Peat-based inoculants can vibrate off the seed if they are not applied with an adequate sticking agent. Liquids could be calibrated poorly and may not hit all the seed if the application is not made carefully. Even use of granular inoculants may have problems in performing if the seeding equipment is poorly calibrated.
If proper care in handling and application is conducted, the success rate of the use of all inoculation is very high. In the rare event that nodulation
does not take place, supplemental N will have to be applied to reach yield potential. In-season foliar N application is not recommended and slow-release liquid N sources have no greater foliar N efficiency, compared with UAN (urea-ammonium nitrate solutions) or urea solutions.
The best inoculation strategy for fields that will be seeded to soybeans for the first time is double-inoculation:usually a peat or liquid seed treatment together with a granular inoculant application.
If a field has been seeded to soybeans previously and nodulation was effective, the chances that inoculating again will be economically effective are small.
Studies in North Dakota have compared many inoculums brands in fields where soybeans previously were grown and successfully inoculated. Only very small yield benefits to inoculation of soybeans on land previously grown to soybean have been seen.
In more than 16 trials from Wishek to Harvey from 1999 to 2018, the average yield increase due to inoculation on land previously grown to soybeans was 2% (Table 1). In a survey of Midwest university soybean inoculation studies (Bly and Gelderman, unpublished data), only 7% of sites with a previous soybean history benefited from inoculation, while 93% of sites without a previous soybean history benefited from inoculation. Locally, NDSU studies indicate that if soybeans had nodules in a field four years in the past, the chances of yield benefits to inoculation are very small.
Studies were performed by NDSU scientists at the Carrington and North Central Research Extension Centers to determine how many Bradyrhizobium bacteria per gram of soil are needed for adequate nodulation and yield. Fields with no history of soybeans typically had zero to 10 of these bacteria per gram, and fields where soybeans had been grown once before typically had 150 to 250 of these bacteria per gram. The data, shown in Figure 3, indicate that after Bradyrhizobium becomes established in the soil, very little if any response to inoculation occurs.
Factor | Number of trials | Option A | Option B | Percent yield A > B |
---|---|---|---|---|
Tillage System | 37 | Reduced tillage | Conventinal tillage | 4 |
Inoculations with soybean history | 16 | Yes | No | 2 |
P application at seeding | 7 | Braodcast | Band away from seet | 0.5 |
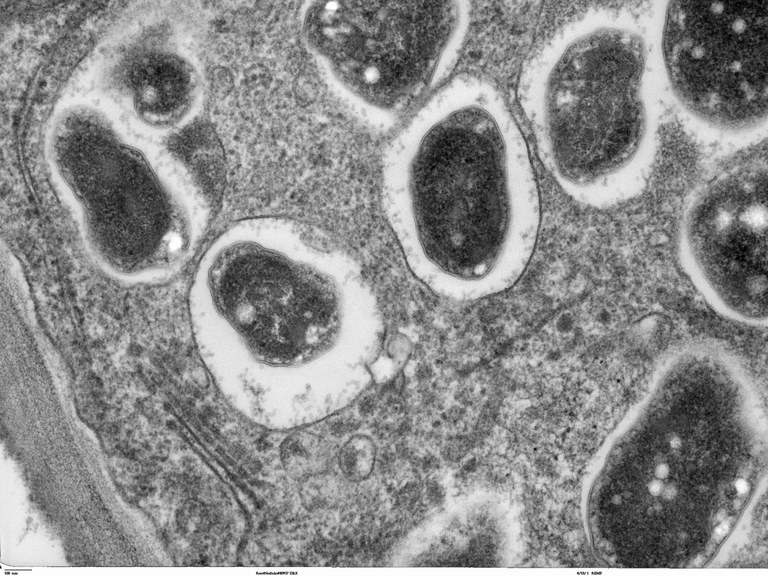
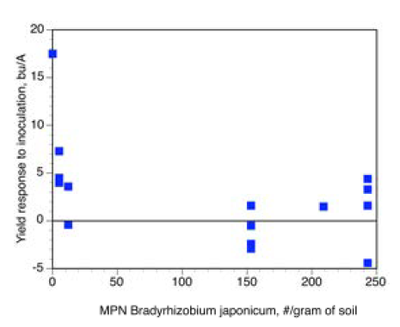
A long-term cropping systems trial at Carrington has included soybeans in a four-year rotation for about 30 years. Soils were sampled one year, two years, three years and four years after the last planting of soybeans. The results showed that where soybeans have been grown for many years, large numbers of Bradyrhizobium remain in the soil, even four years without growing soybeans.
Thus, inoculation of soybeans probably needs to be done only for the first few times that soybeans are grown. Even four years away from soybeans, more 2,000 of these bacteria were found per gram of soil (Table 2), far greater than what was needed, as shown in the prior figure.
Time from last Soybean Planting | Bradyrhizobium Cells per gram soil |
---|---|
1 year | 19,534 |
2 years | 3,718 |
3 years | 2,464 |
4 years | 2,234 |
The number of nodules supported by the soybeans is not related to N availability to the soybeans. Work at the NDSU Carrington Research Extension Center indicates that as long as at least one nodule is on the root, maximum yield should be achieved (Figure 4).
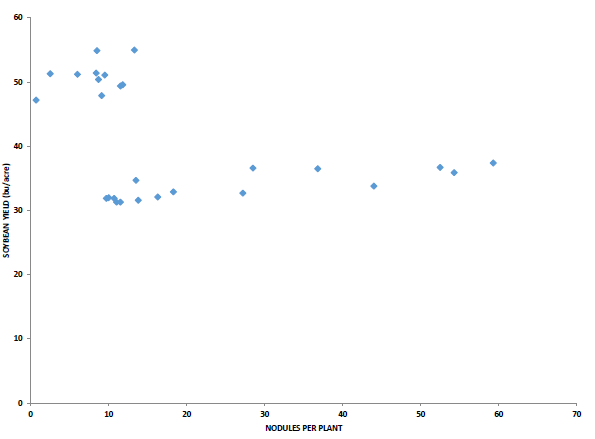
Nitrogen is not required by soybeans if adequate inoculation is present. In North Dakota experiments, the only profitable responses to supplemental N have been to first-year soybeans where initial inoculation resulted in poor nodulation (8) (Table 3).
In a recent study in the Red River Valley of North Dakota (9), the average yield increase of inoculated soybeans, or soybeans in fields with a recent history of soybean cultivation, with an application of 50 pounds per acre of fertilizer N was 1.7 bushels per acre. The value of the soybean yield increase did not pay for the N fertilizer applied.
If soybeans were $10 per bushel and N was 40 cents per pound of N, the application would result in a $3 per acre loss. A 2015-2018 study in northwestern and north-central North Dakota at six sites had no yield increase with 50 pounds per acre of fertilizer N.
Treatment | Yield, bu/acre |
---|---|
Untreated | 21.9 |
50 lb. N/acre, urea | 25.9 |
100 lb. N/acre, urea | 34.5 |
50 lb. N/acre, UAN | 29.0 |
100 lb. N/acre, UAN | 32.9 |
LSD 5% | 6.2 |
Phosphorus
North Dakota soils are typically low in phosphorus (P). The soil test method used to support NDSU recommendations is the Olsen sodium bicarbonate extraction method (10). The Olsen test best predicts crop response in soils within state boundaries that range from a pH below 5 to more than 8.
More than one-half of soils within North Dakota have a pH greater than 7.
Soybean yield responds positively to P application if soil tests are low. Many soybean fields are seeded using grain drills and air-seeders to plant narrow-row soybeans, and many growers would prefer to apply P fertilizer with the seed. Higher soybean yields in medium to low P soils in Minnesota have been reported with P fertilization, whereas yield increases with P application in North Dakota have been small to nonresponsive (Table 4).
Where North Dakota yields have been improved with P, broadcast P application is generally superior to banded P. Part of the advantage of broadcast vs. banded P may be due to stand reduction in seed-placed P application experienced in nearly all North Dakota studies (Tables 5 and 6). Banded P is most successful if separation occurs between the fertilizer band and the seed (Table 7).
In North Dakota, while seed-placed or near-seed placed P application usually is profitable in small grains, canola, corn and sugarbeets, profit is unlikely in soybeans (Table 6). No fertilizer P should be applied with the seed because stand reduction will overwhelm any yield benefit of P application (Table 4).
In the central U.S. Corn Belt, P fertilizer commonly is applied to corn only in a corn-soybean rotation. This practice tends to provide good benefits to soybeans and corn because the soil P levels are most often in the “high” range. In North Dakota, the P test is much lower, so P should be applied to soybeans in a separate application. Fertilizing each crop is important until the field P test reaches the high availability range (Table 8).
P Fertilization* Seed-placed |
P Fertilization* None |
---|---|
37.2 | 40.9 |
* In-furrow rates: 45-50 pounds per acre of 11-52-0 or 4-8 gallons per acre of 10-34-0. |
Rate of 11-52-0 with the seed lb./acre | Final soybean stand, plants per square foot | Yield bushels per acre |
---|---|---|
0 | 3.1 | 38.5 |
45 | 2.3 | 38.3 |
91 | 2.1 | 33.6 |
136 | 2.0 | 35.3 |
Application method | Stand 1,000 plants/acre | Yield bu/acre |
---|---|---|
Check | 187.5 | 32.8 |
2 by 2, 4 gal./acre | 188.6 | 33.5 |
In furrow 4 gal./acre | 133.2 | 24.5 |
In furrow 8 gal./acre | 120.6 | 18.9 |
LSD 5% | 16.5 | 4.3 |
P treatment* | Soybean yield, bushels per acre |
---|---|
Mid-row band | 44.4 |
None | 43.4 |
All years, medium P test; 2009-10, 30-inch rows; 2011, 22-inch rows. 2009-10, 4-6 gallons per acre of 10-34-0; 2011, 10 gallons per acre of 6-24-6. |
Phosphate “inoculants” sometimes are promoted to North Dakota soybean growers. The predominant inoculants are formulations of Penicillium bilajii, a fungus developed for commercial use in Canada about 20 years ago. According to the developer, the fungus excretes acid from its hyphae. The acid can release trapped P from “occluded” P in the soil, which is P that is separated from the soil by a carbonate coating that can be present if the soils are high in free calcium carbonate.
If this P is present in soil, up to 10 pounds of P2O5 per acre may be made available during the course of the season. The application of a P inoculant cannot be expected to act as a starter. The P inoculants will not release P in soil with a pH below 7 (11). Six site-years of work at Carrington in soybeans found no significant yield increase from the use of P inoculants (Endres, unpublished 2012).
Recommended rates of P2O5 to soybeans based on soil test results can be found in Table 8.
Olsen Soil Test Phosphorus, ppm | ||||
---|---|---|---|---|
VL | L | M | H | VH |
0-3 | 4-7 | 8-11 | 12-15 | 16+ |
lbs/acre P2O5 | lbs/acre P2O5 | lbs/acre P2O5 | lbs/acre P2O5 | lbs/acre P2O5 |
52 | 26 | 0 | 0 | 0 |
Soil Test Potassium, ppm | |||||
---|---|---|---|---|---|
VL/VL | L/L | M/M | H/M | VH-H | VH/VH |
0-40 | 41-80 | 81-120 | 121-150 | 151-200 | 201+ |
lbs/acre K2O | lbs/acre K2O | lbs/acre K2O | lbs/acre K2O | lbs/acre K2O | lbs/acre K2O |
90/90* | 60/90 | 60/60 | 30/60 | 0/60 | 0/0 |
* Rate of K2O in soils with smectite:illite ratio less than 3.5; rate of K2O in soils with smectite:illite ratio greater than 3.5. |
Potassium
Potassium requirements are lower for soybeans than for corn. However, in a corn and soybean rotation, fertilizing for higher nutrient levels is important. Although the critical soil level for K using the 1-N ammonium acetate extraction method is only 100 to 150 parts per million (ppm) for soybeans, depending on clay species, the critical level for K in corn may be as high as 200 ppm. Therefore, adding K to replace what the soybean crop may remove will make fertilizing corn less economically and logistically painful the year prior to corn.
In addition, rates of 0-0-60 (muriate of potash K fertilizer) greater than 200 pounds per acre have resulted in lower corn yield, compared with the 200 pounds per acre rate at responsive sites. Therefore, adding some K in the soybean year may improve corn yields the next year if the soil will respond to a buildup in soil test K values.
Very sandy soils will not build K values due to their low cation exchange values. Recommendations for K fertilization of soybeans based on soil test results can be found in Table 8.
In-season Application of Foliar Sprays of N, P and K
Soybean plant physiologists have reported that soybean nodule sustenance by the host plant is reduced soon after pod initiation. The reduction of nodule activity after pod initiation has prompted some to market foliar fertilizer to supplement the reduced nodule activity.
Foliar fertilizer trials have been conducted in soybean-growing areas for the past 40 years. Yield responses to foliar-applied single nutrients or combinations of nutrients have not resulted in consistent yield responses (12, 13). Occasionally a yield response is recorded, but the great majority of experiments and on-farm trials have resulted in no yield increase and sometimes a yield decrease was recorded.
Some of the uncommon yield increases to foliar NPK applications have been related to low availability of soil nutrients. The rest of the yield increases are unexplained. The frequency of yield increases in all studies is so low and so unpredictable that foliar application of NPK to soybeans is not recommended as an economically profitable management practice.
Recent experiments in northwestern and north-central North Dakota during three years, with six total site-years and four annual foliar treatments, showed no yield increases from in-season foliar fertilizer application, and in one year, yield decreased with the application.
Sulfur
Soybeans require sulfur (S) for normal growth and development; however, soybeans appear to utilize and obtain available S better than canola, small grains and corn. The S soil test poorly predicts the need for supplemental S.
A better S fertilization strategy would be to know the local soils and consider past precipitation to determine whether S might be needed each season. If the soils are loam or coarser in texture, and the fall season was wet, snowfall was normal to above normal and/or spring was wet prior to planting, the application of 10 pounds of S as a sulfate form (ammonium sulfate or gypsum) might be helpful.
Elemental sulfur of any formulation is not a recommended S source in North Dakota due to the poor S oxidation rates by microorganisms in the region to plant-usable sulfate. Several recent S rate studies on soybeans in North Dakota indicate that soybean yields would not be improved by S application in North Dakota except under the extreme conditions of low organic matter (less than 2%), coarse-textured soils and excess precipitation.
Iron
About 5% by weight iron (Fe) is in North Dakota soils. However, only a tiny fraction is ever available to plants. Iron in well water is reduced iron (Fe++ or ferrous iron). Ferrous iron is very soluble in water. The weight of a No. 2 carpenter nail can be dissolved in water if it were ferrous iron.
Unfortunately, as soon as ferrous ion is exposed to oxygen, it oxidizes to oxidized Fe (Fe3+ or ferric iron). Ferric iron is a trillion times less soluble than ferrous iron. Plants, except for aquatic plants such as rice and pondweed, implement Fe uptake strategies to improve Fe nutrition and avoid deficiency.
In soybeans, Fe is mobile in the plant from germination through the first mono-foliate leaf. As the first trifoliate leaf emerges, Fe becomes immobile in the plant and must be taken up continually through the season to avoid deficiency.
The soybean strategy for Fe uptake begins by soybean roots acidifying the soil environment directly around the soybean root. An acidic soil environment is necessary for the activity of an Fe-reducing protein that the soybean root secretes (13). If the root remains acidic, the Fe-reducing protein contacts oxidized iron and reduces it to soluble ferrous iron, making it available to the plant.
In soils that support iron deficiency chlorosis (IDC), the causal soil condition is the presence of soil carbonates (CO3-2-) (14). As the soil becomes wetter, the amount of carbonates that are dissolved increases, producing the bicarbonate anion (HCO3 -) (15). Bicarbonate neutralizes the acidity around plant roots and makes the Fe-reducing protein secreted by the roots ineffective (16, 17).
Iron foliar sprays generally are not effective in correcting a deficiency (18). The best application to reduce IDC is ortho-ortho-EDDHA Fe chelate applied with water in-furrow at seeding. The ortho-ortho-EDDHA not only succeeds in delivering Fe to the plant root early in the season, but it also has the ability to go back into the soil solution, bind to additional Fe and deliver it to the plant root with the soil water stream (19).
The ratio of ortho-ortho EDDHA in relation to ortho-para EDDA (Figure 5) in an Fe-EDDHA fertilizer is very important. Recent research at NDSU has shown that the response of soybeans to EDDHA fertilizer is directly proportional to the percentage of ortho-ortho EDDHA Fe (Figure 6).
An effective IDC prevention strategy should not rely on the application of ortho-ortho-EDDHA alone, but on a comprehensive approach to the condition. An IDC-tolerant variety should be selected. A recent four-state study led by NDSU found that the highest yield for a soybean field with soils with and without susceptibility to soybean IDC would be best managed by seeding a high-yielding IDC-susceptible cultivar in non-IDC soils and an IDC-tolerant cultivar in the IDC-susceptible soils (20).
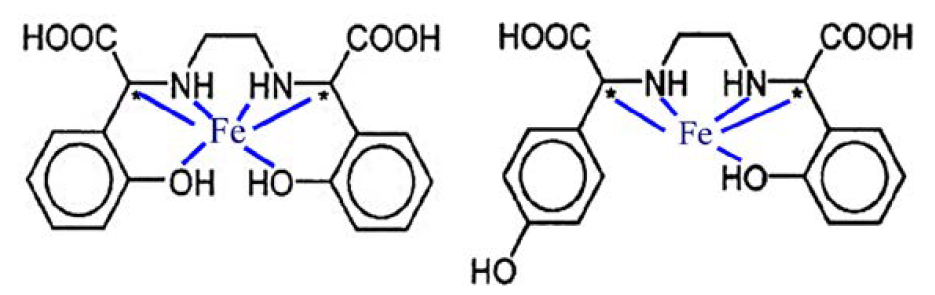

To reduce IDC pressure, soybeans should be seeded in rows wider than 15 inches if wider rows will not result in difficult weed control problems. Although the exact mechanism for denser stands is not known, many growers have seen this effect when the planter stops within the field and leaves a high strip of seed behind when it resumes planting.
Soybeans in densely seeded areas are taller and have less IDC symptoms, compared with the normally seeded fields. Similar reduction in IDC symptoms are seen as soybeans are seeded closer to each other in wider row spacings or higher seeding rates (21). The reasons for IDC reduction with denser seeding might be related to reduced soil moisture under the row, or greater root-zone acidity that favors activity of the Fe-reducing soybean root secretions.
A three-state study found that seeding a cover crop of 1 bushel per acre of oats or other easily killed small-grain cover crop in the soybean field near the date of soybean planting can reduce excess water and take up some excess soil N (Figures 8a and 8b, Page 9). Depending on soil moisture, the oats may be killed with herbicide early if conditions are dry, or up to the five-leaf stage of oats if the season is wet. The use of an oat cover crop resulted in as high as 40 bushels per acre more soybeans where oats were used, compared with no inter-crop at a Minnesota site in a wet season (7) (Table 9).
Because soil salinity aggravates and increases the severity of IDC, a comprehensive, rotation-based strategy should be imposed to reduce soil salinity as much as possible. The strategy should include the selection of salinity-tolerant crops; use of alfalfa strips to reduce roadside salinity; use of alfalfa above saline seeps to reduce the severity of the seep; use of cover crops when possible before, during or after cropping to reduce field water table; and tile drainage if possible, practical and socially and/or regulation permissible.
N applied pounds per acre | 0 | 100 | 200 | 0 | 100 | 200 |
---|---|---|---|---|---|---|
Oats | No | No | No | Yes | Yes | Yes |
C06* (bushels per acre) | 42.1 | 28.6 | 25.3 | 42.5 | 20.5 | 18.9 |
YM06 (bushels per acre) | 52.0 | 32.2 | 19.1 | 52.4 | 42.6 | 25.9 |
K07 (bushels per acre) | 3.7 | 0.3 | 0.1 | 40.2 | 24.5 | 7.2 |
YN07 (bushels per acre) | 51.7 | 46.5 | 40.2 | 50.7 | 43.4 | 33.7 |
C08 (bushels per acre) | 34.3 | - | - | 41.7 | - | - |
R08 (bushels per acre) | 30.4 | - | - | 28.1 | - | - |
C09 (bushels per acre) | 51.0 | - | - | 50.0 | - | - |
R09 (bushels per acre) | 42.0 | - | - | 44.0 | - | - |
* Headings indicate different sites/years of study. |
A pictorial representation of the IDC ratings for soybeans used by the NDSU soybean breeder and scientists can be found in Figure 7.
The strategy for IDC management should be built around the following points:
- Field selection – In the east, where IDC is prevalent, select fields with lower carbonate and EC values.
- Select varieties that are tolerant to North Dakota IDC. Relative IDC values can be found in NDSU variety trial summaries from NDSU Research Extension Centers. The summaries are published each year.
- Increase the seeding rate and grow in wider row widths if practical.
- Use an oat/barley companion crop at/near seeding.
- Apply an Fe-ortho-ortho-EDDHA fertilizer with the seed at planting.
A long-term strategy to reduce IDC in the east through time should include the reduction of salinity (EC) through improved drainage and better crop selection to lower soil water table depth.
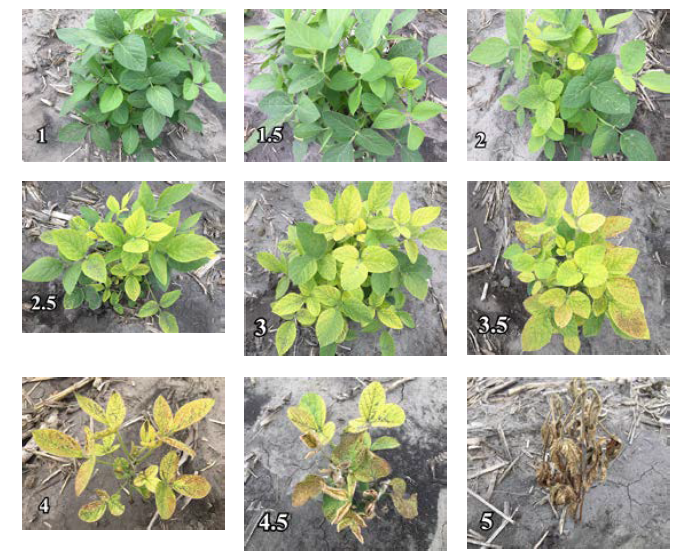
Other Nutrients
Deficiencies of zinc, manganese, boron, molybdenum, nickel, chloride or copper have not been recorded in North Dakota. Field experiments have not revealed any supplemental requirement for these nutrients above what our soils currently provide. A recent study that includes cobalt, an essential element for N-fixing bacteria, found no yield or protein increase due to its application.
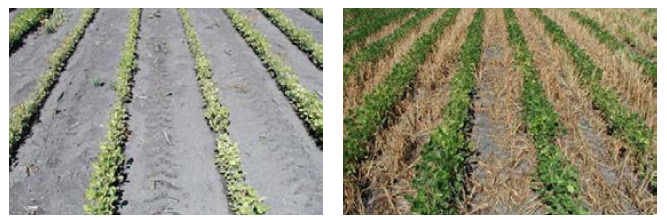
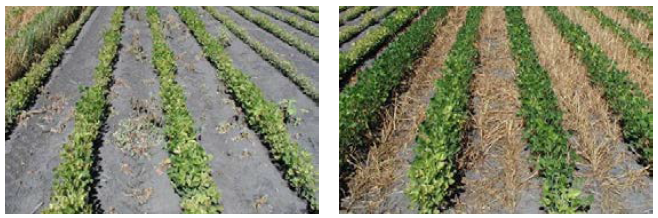
Soil pH and Liming
Recent soybean fertility studies have investigated lime application on acid soils (22). The lowest pH in these studies was 5.7, and no yield improvement occurred due to lime application at any location. The “critical” soil pH for soybeans published in Corn Belt states is about 6.5. Most North Dakota subsoil pH values likely are higher than the surface pH, and that is why lime application in these studies did not increase soybean yield.
In no-till fields with an acid pH, the best option is to sample the field by zone at the zero to 2-inch depth and the 2- to 6-inch depth. Western North Dakota has growing areas with aluminum (Al) toxicity problems caused by surface soil pH levels less than 5.2.
Soybeans would be susceptible to Al toxicity. Therefore, keeping the soil pH, particularly at the surface, greater than pH 5.6 would be recommended. In loam soils, 1 to 2 tons per acre of sugarbeet lime, city water treatment lime or good-quality calcitic or dolomitic limestone would be enough to increase surface pH at least 0.5 unit.
References
- Subramanian, S., G. Stacey and O. Yu. 2007. Distinct crucial roles of flavenoids during legume nodulation. Trends in Plant Science 12:282-285.
- Stacey, G., M. Libault, L. Brechenmacher, J. Wan and G.P. May. 2006. Genetics and functional genomes of legume nodulation. Current Opinions in Plant Biology 9:110-121.
- Oldroyd, G.E.D., and J.A. Downie. 2008. Coordinating nodule morphogenesis with rhizobial infection in legumes. Annual Review of Plant Biology 59:519-546.
- Lamb, J.A., G.W. Rehm, R.K. Severson and T.E. Cymbaluk. 1990. Impact of inoculation and use of fertilizer nitrogen on soybean production where growing seasons are short. Journal of Production Agriculture 3:241-245.
- Goos, R. Jay, Shana Forster, Jasper M. Teboh, Gregory Endres and Ezra Aberle. 2018. Field calibration of the ureide and MPN tests for adequacy of nitrogen fixation by soybean. Proceedings of the Great Plains Soil Fertility Conference, Denver, Colo.
- Dose, H.L. 2016 Advancing soil health: Linking belowground microbial processes to aboveground land management. NDSU Ph.D. thesis.
- Bloom, P.R., G.W. Rehm, J.A. Lamb and A.J. Scobbie. 2011. Soil nitrate is a causal factor in iron deficiency chlorosis in soybean. Soil Science Society of America Journal 75:2233-2241.
- Endres, G., E. Aberle and R. Henson. 2002. N-deficient soybean response to POST N, Cathay, 2002. In Carrington Research Education Center Annual Report. NDSU Agricultural Experiment Station Report.
- Buetow, R. 2015. Nitrogen management in dry bean and soybean. MS thesis, NDSU, Fargo, N.D. https://library.ndsu.edu/ir/bitstream/handle/10365/27835/Nitrogen%20Man… (accessed July 2019).
- Frank, K., D. Beegle and J. Denning. 1998. Phosphorus (revised January 1998). In: Chapter 6, Recommended chemical soil test procedures for the North Central Region. North Central Regional Research Publication No. 221 (Revised). University of Missouri, Columbia, Mo.. Missouri Agricultural Exp. Station SB 1001. http://extension.missouri.edu/explorepdf/specialb/sb1001.pdf (accessed July 2019).
- Goos, R.J., B.E. Johnson and R.W. Stack. 1994. Penicillium bilajii and phosphorus fertilization effects on the growth, development, yield and common root rot severity of spring wheat. Fertilizer Research 39:97-103.
- Haq, M.U., and A.P. Mallarino. 1998. Foliar fertilization of soybean at early vegetative stages. Agronomy Journal 90:763-769.
- Mallarino, A.P., M.U. Haq, D. Wittry and M. Bermudez. 2001. Variation in soybean response to early season foliar fertilization among and within fields. Agronomy Journal 93:1220-1226.
- Schmidt, W. 1999. Mechanisms and regulation of reduction-based iron uptake in plants. New Phytologist 141:1-26.
- Franzen, D.W., and J.R. Richardson. 2000. Soil factors affecting iron chlorosis of soybean in the Red River Valley of North Dakota and Minnesota. Journal of Plant Nutrition 23:67-78.
- Zocchi, G., P. De Nisl, M. Dell-Orto, L. Espen and P.M. Gallina. 2007. Iron deficiency differently affects metabolic responses in soybean roots. Journal of Experimental Biology 58:993-1000.
- Bloom, P.R., and W.R. Inskeep. 1986. Factors affecting bicarbonate chemistry and iron chlorosis in soils. Journal of Plant Nutrition 9:215-228.
- Chatterjee, A., S. Lovas, H. Rasmussen and R.J. Goos. 2017. Foliar application of iron fertilizers to control iron deficiency chlorosis of soybean. Crop, Forage & Turfgrass Management. Volume 3. doi:10.2134/cftm2017.05.0037
- Lovas, S.E.H. 2013. Laboratory and greenhouse evaluation of FeEDDHA fertilizers of differing quality. North Dakota State University, Department of Soil Science MS thesis. https://library.ndsu.edu/ir/bitstream/handle/10365/27101/Laboratory%20a… (accessed August 2019).
- Helms, T.C., R.A. Scot, W.T. Schapaugh, R.J. Goos, D.W. Franzen and A.J. Shlegel. 2010. Soybean iron-deficiency chlorosis tolerance and yield decrease on calcareous soils. Agronomy Journal 102: 492-498.
- Goos, R.J., and B. Johnson. 2001. Seed treatment, seeding rate and cultivar effects on iron deficiency chlorosis of soybean. Journal of Plant Nutrition 24:1255-1268.
- Franzen, D.W., and C. Augustin. 2019. Soybean soil fertility in northwest and north central North Dakota. Project Ending Report to the North Dakota Soybean Council, Fargo, N.D.